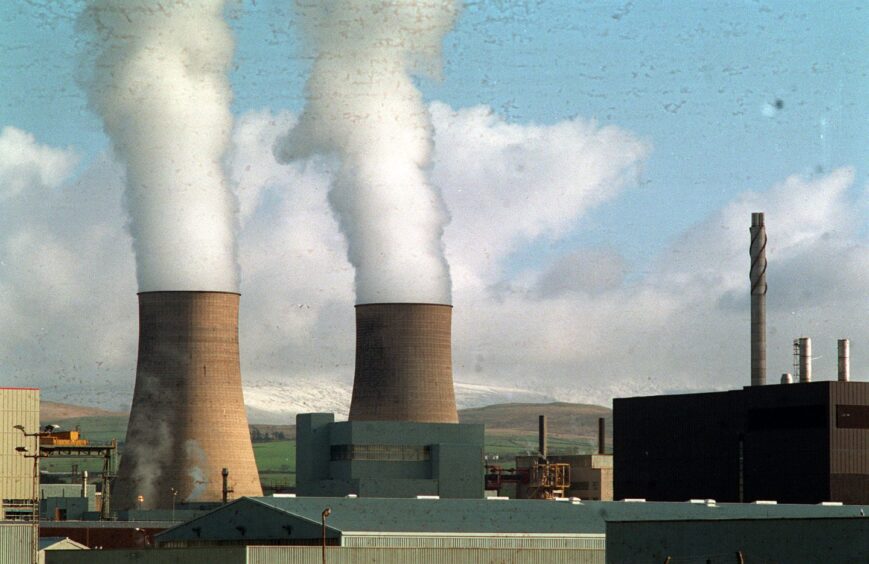
As a child, my father, a senior experimental plasma physicist at the UK’s Culham Laboratory, would tell me that an electricity-generating fusion reactor was just 30 years away. His opinion had not changed by the time he retired, and I believe it would be the same now, if he were alive. But then, he always was an optimist.
With the exception of those on which their business is based, such as France’s EdF, electric utilities in the western world have largely given up on building new nuclear fission reactors. They are expensive; the capital outlay and commercial risks are too high, and they take too long to build.
Market forces and climate policies are now driving the construction of wind and solar farms, which generate cleaner electricity more cheaply, even when energy storage is included. As nuclear power has largely failed as a commercial market proposition, nearly all nuclear newbuild in the world today is heavily state sponsored in one form or another, rather than market driven.
But the nuclear industry is far from out. It has ‘new’ propositions, one of which is still nuclear fusion.
Dubious claims
Private companies have entered the sector, claiming that they can solve the problems encountered by decades of international research with new reactor designs and fusion processes.
Investors hope that innovation from an agile private sector will rejuvenate and overtake the slow process of publicly funded science, represented by the ITER project currently under construction at Cadarache, in France. Fusion will generate limitless clean energy and, in the process, become a key tool for addressing climate change – according to its proponents.
US company Helion, which in 2015 promised a “a useful reactor in the next three years”, now promises a fusion plant by 2028, for example. Microsoft has even agreed to purchase electricity from the facility.
However, the claims of clean, unlimited energy do not stand up to scrutiny. Or as nuclear fusion scientist SJ Zweben put it more bluntly in an article for Physics and Society in January, they are:
“At best wildly optimistic but more often mistaken, delusional, deceitful or fraudulent.”
The scientific and engineering challenges facing nuclear fusion reactors are legion, and as Zweben points out they all need to be resolved at the same time. This is extremely challenging because the solutions proposed for one problem often exacerbate others or create new ones.
The many challenges include energy confinement, impurity contamination, plasma disruptions, wall erosion, the tritium fuel cycle, availability in terms of operational uptime, excessive power consumption by the plant itself, cost and – yes, contrary to industry marketing – radioactive waste.
Spherical Tokamak for Energy Production (STEP)
The UK is basing its fusion hopes on STEP, having left the international ITER project with Brexit. A site has been chosen for the project, but it is not yet clear whether the experiment will garner the same support from the current government as it did from the previous one.
In a recent article for Physics World, fusion scientist Guy Matthews noted that the energy stored in STEP’s plasma would need to be about 5,000 times larger than that produced in the UK’s MAST-U spherical tokamak experiment. He describes the single giant leap to a power plant as “an extreme, and unprecedented, extrapolation of physics and technology”.
It may even be dangerous. There is no way yet of reliably avoiding or mitigating plasma instabilities, known as ‘disruptions’. Without a robust solution, the consequent damage “would render a power plant inoperable”.
Other experienced fusion scientists share these and other concerns.
John Evans, who worked at the Atomic Energy Research Establishment in Harwell, recently highlighted the lack of a proven solution for the fusion fuel cycle.
This involves breeding and reprocessing unprecedented quantities of radioactive tritium – a hydrogen isotope that does not occur naturally and needs to be generated from a massive ‘breeding blanket’ containing lithium. A solution must be in place before any fusion power plant can operate and each fusion plant would consume, annually, more tritium than is currently available globally.
Put simply, the technical and scientific challenges posed by any approach to fusion, whether using spherical, ‘toroidal’ tokamaks or lasers, are huge.
Will fusion be clean?
According to the International Atomic Energy Agency (IAEA), “Fusion does not create any long-lived radioactive waste”. This is true only in theory.
- A fusion reactor produces helium, an inert gas, as a result of a fusion reaction between the hydrogen isotopes tritium and deuterium. Tritium is very radioactive with a half-life of 12.3 years. The tritium is both produced and consumed by the fusion reactor so, in a perfect world, there is no nuclear waste.
- However, 80% of the power from the fusion reaction is delivered as fast neutrons that generate the tritium from the surrounding breeding blanket, which is likely to require periodic replacement.
- Nuclear reactions between the neutrons, and impurities or primary elements in the blanket, make it radioactive and degrade the materials – i.e. increasing the need for replacement.
- Materials in a more compact fusion reactor, like STEP, would accumulate neutron damage more rapidly and would therefore need more frequent replacement.
As a result, Matthews provides a somewhat different message to the IAEA: “If conventional engineering materials are used, fusion reactors have the potential to generate far larger volumes of long-lived radioactive waste than fission reactors.”
The extent to which suitable low-activation fusion materials can be developed to mitigate this challenge at an acceptable cost is one of the many unsolved problems facing fusion power.
A neutron-free fusion reaction is possible using hydrogen and boron, but for this to work the plasma temperature needs to be around 7,000 million degrees – which makes the deuterium-tritium reaction (JET, ITER), at a mere 100 million degrees, seem like a walk in the park.
Fusion’s costs are misunderstood or ignored
Fusion advocates use the term ‘limitless’ energy to imply cheap energy. But will fusion provide either?
It could be limitless in the sense that the base fuel sources – lithium and deuterium – are abundant and only relatively small amounts are required to produce huge amounts of power. Unfortunately, the idea that a limitless or near-limitless energy source means cheap energy is plain wrong because, however energy is generated, it has a cost.
A nuclear fusion power plant will have a capital cost, an operational cost and a maximum generating capacity like any other power plant. The price of a first-of-a-kind reactor will be huge and an ‘nth of a kind’ reactor will not be cheap. ITER’s costs are currently estimated at €18-22 billion, but will likely prove much higher and it is an experiment – not a power plant.
STEP’s cost is estimated to run to several billion pounds before construction has even started and it is a far more challenging project. Moreover, the role of STEP (if successful) is only to provide a “pathway to commercialisation” according to Howard Wilson, fusion pilot plant lead at the US’ Oak Ridge National Laboratory.
Cost trajectory
For wide-scale deployment, fusion must be economically viable. The general ‘rule’ used in forecasting future costs is that they halve as production of a commodity doubles. However, this is a popularisation and over-optimistic simplification of Wright’s Law, which states that for every cumulative doubling of units produced, costs will fall by a constant percentage.
The extent of that percentage is usually governed by the complexity of the technology concerned and the degree to which it can be modularised and subject to the cost gains of mass manufacturing. Technical complexity and safety concerns, when major, mean that the cost reduction of higher production volumes can be small or non-existent.
Just as nuclear fission has struggled to follow Wright’s Law, there is no reason to believe that fusion, which is much more complex, will be any more successful.
Relevance to climate change
Nuclear fusion is still decades away from working (i.e. producing sustainable net energy gains), and then decades more from economic viability. Even then, it would be more decades still from deployment on a scale large enough to have any impact on climate change.
It is almost 2025, and to remain on track to limit global warming to 1.5°C above pre-industrial levels, the world, not just individual countries, needs to achieve net-zero carbon by 2050. It is a goal that is already slipping away. Fusion is simply too far off to be of any use.
Ross McCracken is a freelance energy analyst with more than 25 years experience, ranging from oil price assessment with S&P Global to coverage of the LNG market and the emergence of disruptive energy transition technologies.
Recommended for you
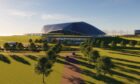