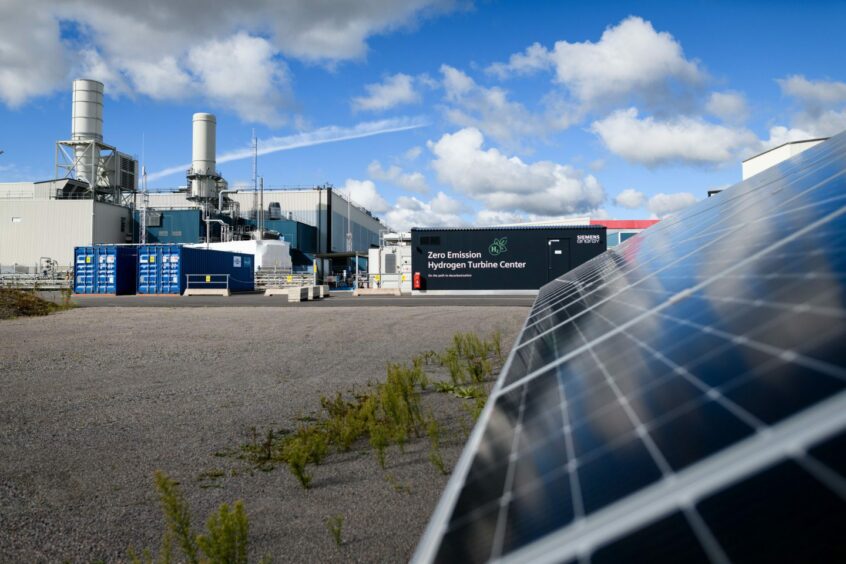
The challenges to making green hydrogen production commercially viable can be tackled if the right technology and economic infrastructure are in place, writes Maria Anez-Lingerfelt, senior scientist at Pall Corporation.
The demand for new green energy strategies is increasing and hydrogen is regarded as a key factor in meeting international emissions targets and achieving net zero by 2050. The UK government has ambitions to double production of hydrogen to 10GW by 2030, and the US’ Department of Energy has announced plans to fund a $8bn programme of regional clean hydrogen hubs to connect producers and consumers with infrastructural frameworks.
In the EU, the REPowerEU plan sets out a vision for the bloc to produce 10 million tonnes and import 10 million tonnes of renewable hydrogen by 2030 – nearly double the figures from the 2021 revised Renewable Energy Directive. While in Saudi Arabia, Crown Prince Mohammed bin Salman wants his country to be the world’s biggest exporter of hydrogen when a new plant opens at the Neom city project in 2026.
In industry, oil and gas giants are also ramping up investment in green energy production. BP is paying $36bn for a 40.5% stake in the Asian Renewable Energy Hub, set to be one of the largest green hydrogen and renewable energy hubs in the world. Shell has committed to building a renewable hydrogen plant in the Netherlands, which will be the largest in Europe once operational in 2025. China National Petroleum Corp has pledged to move 50% of its output to hydrogen, geothermal energy, and clean power by 2050. While Exxon Mobil is planning to build a $100bn hydrogen production and carbon capture plant in Texas.
The global energy transition from fossil fuels to renewables and low carbon power generation is progressing, but there are challenges.
Meeting the challenges
International classification society DNV has produced its first standalone hydrogen forecast, saying that hydrogen is likely to account for 11% of Europe’s energy mix by 2050, but only 5% globally. It states that hydrogen is expensive and inefficient to produce, compared to direct electrification, but notes that its use will be vital for aviation, shipping and high-heat industrial processes which are difficult to electrify.
So, what are the options? Of the different ‘colours’ of the gas, grey and brown hydrogen are produced from fossil fuels and emit carbon dioxide, which needs to be captured and stored to reduce its polluting effects on the environment. If a carbon capture step is added, then it will be termed blue hydrogen.
Green hydrogen is made by the electrolysis of water powered by renewable energy sources; it does not produce CO2 and so is the ‘cleanest’ option. To make green hydrogen commercially viable at scale, there will need to be increased power capacity from wind farms and solar plants and the optimisation of the production process using electrolysers to carry out the chemical process.
Optimising the green hydrogen production process
The electrolysis process involves the dissociation of the water molecule in an electric field. Hydrogen is then produced at the cathode and oxygen at the anode with an electrolyte present in between the electrodes.
There are three types of electrolysers that can be used. The alkaline electrolyser (AEL) uses liquid potassium hydroxide as the electrolyte and is the most widely used in industrial applications, but comes with some drawbacks, including lower purity levels and lower energy efficiency. Polymer electrolyte membrane electrolyser (PEM) uses a solid polymer electrolyte and is increasingly being favoured because it has fewer of these drawbacks but is still expensive compared to AEL. Solid oxide electrolysers (SOEC) use a solid ion-conducting ceramic electrolyte – a technology that holds great potential but is still in early stages of commercialisation.
Whichever electrolysis technology is used, the hydrogen stream needs to be further processed to remove solid, liquid, and gaseous contaminants. It is in this process that the real technical challenge lies because the regulations around gas purity specifications are extremely stringent. Typically, concentrations between 2,000-6,000 ppm (parts per million) of oxygen and more than 2,000 ppm of water are seen contaminating the hydrogen produced using commercial alkaline electrolysis. The maximum concentration allowed for fuel cell vehicles is 5 ppm of each under the ISO standard for hydrogen fuel quality.
Filtration to ensure purity
This gap between outputs and purity standards is why AEL systems – the most widely used in industrial applications – require further optimisation to produce green hydrogen at a large scale. Several unit operations using filtration, separation and purification technology are needed to achieve the required purity levels.
Once the hydrolysis has taken place, the liquid/gas mixture needs to be cooled, separated, and compressed. However, cooling the gas has major cost implications and can be as high as 5% of the total system outlay. Gravity separators, mist eliminators pads, filter vane separators and more recently liquid/gas coalescers are used to separate the liquid contaminants. These typically require large housings and must be operated at low velocities to prevent liquid re-entrainment. After removing the liquids, the hydrogen is fed into a catalytic reactor to remove the contaminating oxygen. The catalyst in this step is sensitive to contamination such as potassium hydroxide (KOH) so the hydrogen entering this step must meet the required purity specifications.
To add to the purification costs, solid contaminants, originating from oxidation in process piping and equipment such as pumps and compressors, must be eliminated. Adsorbent fines – if being used – are situated in the final drying equipment and may also get released, contaminating the gas. To remove the solid contaminants, disposable gas filters in different micron ratings can be deployed throughout the process.
The final step is the efficient storage of the hydrogen once it is produced. It can be compressed and stored in tanks, pumped into salt caverns, or converted into liquid ammonia, using the Haber-Bosch Process. Throughout each of these processes the gas can become contaminated with liquids, solids, or gases (if transported in natural gas pipelines) and the contaminants will need to be removed to meet the end use specifications.
Collaboration is key to the green hydro dream
The Energy Transition Institute’s hydrogen maturity curve notes that green hydrogen is more expensive to produce than brown hydrogen. Yet with technology already available to commercial producers, the price of production will come down as adoption of large electrolysers increases. A report by BloombergNEF finds that when taking into consideration storage and pipeline costs of fossil fuels, green hydrogen could be cheaper to deliver than natural gas by 2050.
To turn this into reality requires the alignment of several factors. There needs to be more solar, wind and other renewable projects to power the process. Investments in infrastructure to store and deliver green hydrogen at the correct specifications must increase. And perhaps we need more ambitious projects such as with Saudi Arabia’s Neom, where authorities, financial institutions, energy companies and technology suppliers collaborate to make faster progress.
Recommended for you
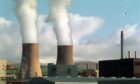