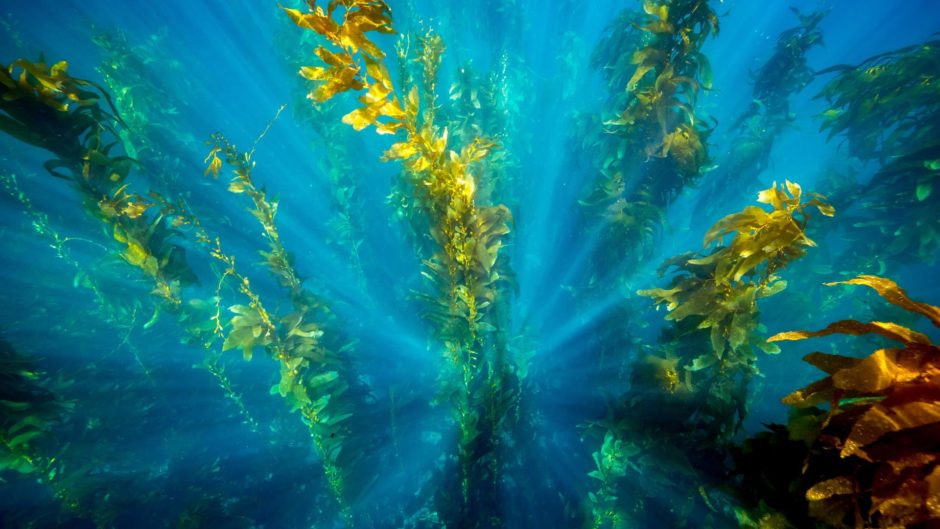
Hydrogen is the most common element in the Universe. On Earth, nearly all chemical fuels are based on hydrogen, albeit in a bound form as hydrocarbons or other hydrogen compounds.
It is the cleanest fuel of all, the residue of combustion being water. But huge challenges stand in the way of large-scale, clean extraction, storage and transportation of the gas.
Numerous projects are under way to crack those challenges and liberate this “holy grail” solution to our mounting energy problems.
In this, the second of our now quarterly round-up of energy R&D, in universities and institutes, we begin with a team at Fraunhofer-Gesellschaft in Germany, who have developed an elegant membrane technology for the efficient separation of hydrogen from natural gas.
How does it work? This is where carbon comes in. It forms an ultrathin layer on porous, ceramic substrates where it separates natural gas and hydrogen.
When the gas mixture reaches the input side of the membrane, the small hydrogen molecules pass through to their destination, while the larger methane molecules are held back.
According to Fraunhofer, the system makes it possible for hydrogen and natural gas to be mixed and transported through an adapted grid and then split up at their final destination. This is a major step forward in the transportation and distribution of hydrogen as an energy source.
Hydrogen is seen as a beacon of hope for establishing a CO2-free energy supply. Offshore wind and photovoltaic powered electrolysers have the potential to produce large quantities of hydrogen cheaply and cleanly.
But how do we move this “green” hydrogen from the producer to the consumer at scale? Germany, for example, still does not have an extensive distribution network for hydrogen. Nor does the UK.
However, it is possible to transport a percentage of hydrogen (up to 20% blend) with natural gas using existing infrastructure.
The Hydrogen Power Storage & Solutions East Germany project is working to solve this dilemma by co-mingling for transportation and distribution by pipeline networks, natural gas and hydrogen, and then separating them at destination.
The aim is to create an intelligent infrastructure of distributor networks and storage stations that will make the clean energy source available to all regions of the former East Germany, at least initially.
The project partners, numbering more than 130, include eight research units or institutes of the Fraunhofer-Gesellschaft, including Fraunhofer IKTS.
SUNLIGHT ELECTROLYSIS
How to produce hydrogen cheaply using electrolysers has exercised minds ever since electrolysis of water was pioneered by William Nicholson (1753-1815) and Anthony Carlisle in 1800.
One approach now being examined involves the use of sunlight.
Researchers at Linkoping University, Sweden, have developed a material, nanoporous cubic silicon carbide, that exhibits promising properties to capture solar energy and efficiently split water for hydrogen gas production.
The potential prize is enormous as hydrogen has an energy density three times that of petrol. It can generate electricity using a fuel cell, and hydrogen-fuelled cars are gradually becoming commercially available.
The Linkoping researchers say that producing hydrogen gas by splitting water molecules with the aid of solar energy is a sustainable approach that could give hydrogen gas using renewable sources without leading to carbon dioxide emissions.
A major advantage of this method is the possibility to convert solar energy to fuel that can be stored.
Conventional solar cells produce energy during the daytime, and the energy must either be used immediately, or stored in, for example, batteries.
Hydrogen can be stored and transported in broadly similar ways to traditional hydrocarbon fuels such as petrol and diesel.
It is not, however, an easy task to split water using the energy in sunlight to release hydrogen gas. For this to succeed, it is necessary to find cost-efficient materials that have the right properties for the reaction in which water is split into hydrogen and oxygen through photo-electrolysis.
The energy in sunlight that can be used to split water is mostly in the form of ultraviolet radiation and visible light. Therefore, a material is required that can efficiently absorb such radiation to create charges that can be separated and have enough energy to split the water molecules into hydrogen and oxygen gases.
Most materials that have been investigated until now are either inefficient in the way they use the energy of visible sunlight, or do not have the properties needed to split water to hydrogen gas.
At Linkoping the scientists have lighted on cubic silicon carbide (3C-SiC) and have developed a form that has many extremely small pores. It turns out that nanoporous 3C-SiC has promising properties that suggest it can be used to produce hydrogen gas from water using sunlight.
Crucially, this new porous material has been shown to efficiently trap and harvest ultraviolet and most of the visible sunlight.
Furthermore, the porous structure promotes the separation of charges that have the required energy, while the small pores give a larger active surface area. This enhances charge transfer and increases the number of reaction sites, thus further boosting the water splitting efficiency.
Will the Swedish team reach their dream of producing copious quantities of hydrogen from water using just sunlight and a catalyst? That’s unknown.
But the project demonstrates how far scientists are prepared to go in their quest to find the hydrogen holy grail.
SOLAR H2 TO SEAWEED!
And now for something completely different — converting seaweed to biofuels.
Biofuel crops are no longer novel. They have even become highly controversial and been fairly extensively investigated by the Norwegians.
However, scientists at the University of Southern California (USC) believe that kelp could be grown, cropped and processed into biofuels.
It already grows at breakneck speed, but the team has developed a system known as the “kelp elevator” that can grow this energy-rich super-algae four times faster.
Scientists at USC’s Wrigley Institute for Environmental Studies say it may be possible to use the open ocean to grow kelp crops for low-carbon biofuel similar to how land is used to harvest fuel feedstocks such as corn and sugarcane — and with potentially fewer adverse environmental impacts.
“Forging new pathways to make biofuel requires proving that new methods and feedstocks work. This experiment on the Southern California coast is an important step because it demonstrates kelp can be managed to maximise growth,” says Diane Young Kim, author of the study.
If it lives up to its potential, kelp is a more attractive option than the usual biofuel crops for two very important reasons.
First, ocean crops do not compete for fresh water, agricultural land or artificial fertilisers.
Second, it is claimed by the California team that ocean farming does not threaten important habitats when marginal land is brought into cultivation.
Giant kelp is one of nature’s fastest-growing plants and its life cycle is well understood, making it amenable to cultivation.
But farming kelp requires overcoming a few obstacles. To thrive, kelp has to be anchored to a substrate and only grows in sun-soaked waters to about 60 feet deep. But in open oceans, the sunlit surface layer lacks nutrients available in deeper water.
To maximise growth in this ecosystem, the scientists had to figure out how to give kelp a foothold to hang onto, lots of sunlight and access to abundant nutrients. And they had to see if kelp could survive deeper below the surface where it is starved of sunlight. So they developed a system known as the elevator.
Beginning in 2019, research divers collected kelp from the wild, affixed it to the kelp elevator and then deployed it off the northwest shore of Catalina Island, near Wrigley’s marine field station.
Every day for about 100 days, the elevator would raise the kelp to near the surface during the day so it could soak up sunlight, then lower it to about 260 feet at night so it could absorb nitrate and phosphate in the deeper water.
The researchers continually checked water conditions and temperature while comparing their kelp to control groups raised in natural conditions.
“We found that depth-cycled kelp grew much faster than the control group of kelp, producing four times the biomass production,” Kim says.
Developing a new generation of biofuels has been a priority for California and the US federal government.
The US Department of Energy’s Advanced Research Projects Agency-Energy has so far invested $22 million in efforts to increase marine feedstocks for biofuel production.
This included $2m to conduct the kelp elevator study.
Not everyone is convinced that bio-cropping kelp is viable, not least at the Bellona Foundation in Norway.
The NGO says there are significant challenges associated with using seaweed for biofuel, including how to reduce local environmental impacts of concentrated culture and harvesting operations.
Growing seaweed could reduce the amount of nutrients in an area, which could affect other sea life higher up the food chain.
Bellona says several potential impacts need further study, such as the effects of more human activity, risks of cross-breeding wild and cultivated species, diseases and the creation of a non-natural temporary habitat (as a seaweed farm would be).
Bellona published an extensive study on seaweed to energy in 2017. It is understood that there are currently 18 different companies that have received a license to operate commercial seaweed plants in Norway in 26 different locations on the west coast from Rogaland to Nordland.